Disturbance of the gut microbial metabolism is thought to be the root cause of human diseases. Bacteria, viruses, and fungi affect their human hosts in numerous ways. There is evidence to support the theory that microbes, through their genetic makeup, gene products, and metabolites, play a role in human physiology, metabolism, immunity, disease susceptibility, and response to pharmacotherapy, as well as the severity of disease-related side effects.
Of more importance to oncologists, the microbiome may significantly affect the tumor microenvironment. Specifically, the richness and diversity of the fecal or gut microbiome appear to affect carcinogenesis, response to antitumor treatment (especially immunotherapy), as well as the severity of immune-mediated adverse effects. These effects have best been described in melanoma, but recent studies in other solid tumors, including colon and lung cancers, have shown compelling results. Recently, oncologists have begun to question whether this research can be applied to gynecologic cancers.
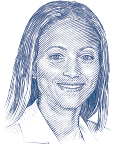
Nathalie D. McKenzie, MD, MSPH, FACOG, DipABLM
Chronic Diseases Alter the Gut Microbiome
It is well known that gynecologic malignancies are influenced by underlying chronic diseases, including obesity (and its associated low chronic inflammation), oncogenic virus infections, and dysregulated hormonal interactions. There is evidence to suggest that gut dysbiosis gives rise to a diverse spectrum of both local and systemic chronic diseases. Dysbiosis of the gut microbiota is, in fact, implicated in autoimmune and chronic inflammatory conditions such as obesity, inflammatory bowel disease, rheumatoid arthritis, Alzheimer’s disease, cardiovascular disease, and nonalcoholic fatty liver disease.
For example, one molecular epidemiologic study showed that there were increased levels of Lactobacillus salivarius, Lactobacillus iners, and Lactobacillus ruminis in patients with rheumatoid arthritis compared with the control group, and the presence of Lactobacillus mucosae was unique to patients with rheumatoid arthritis.1 Scher et al observed that the majority of patients with new-onset, treatment-naive rheumatoid arthritis carry high levels of Prevotella copri, a unique species that defines the microbial genome of these patients.2 Gut Prevotella, a Gram-negative anaerobe, appears to be relevant in many inflammatory and autoimmune conditions.3
The brain-gut-microbiota axis is implicated in Alzheimer’s disease, whose pathogenesis has been attributed to extracellular aggregates of β-amyloid plaques. Specifically, microbial metabolites, such as short-chain fatty acids and tryptophan metabolism by-products, have been shown to play a role in both microglia maturation and astrocyte activity modulation, respectively. The production of amyloids by microbiota and their direct exposure within the gut may cause immune system priming and may amplify the immune response to endogenous neuronal amyloid production. Using molecular mimicry, bacterial amyloids may cross-react with host amyloids to produce a pathogenic β-sheet structure.4
The production of metabolic by-products and variations in gut microbiota taxa both contribute to the development of cardiovascular disease. Microbes metabolize dietary nutrients containing choline, phosphatidylcholine, or L-carnitine into trimethylamine, which then become oxidized in the liver into the proatherogenic metabolite trimethylamine N-oxide.5 In an atherosclerotic cardiovascular-diseased gut, the microbiome was shown to be in dysbiosis with a surplus of Enterobacteriaceae, Streptococcus spp, and others commonly found in the oral cavity, such as Ruminococcus gnavus, a bacteria associated with inflammatory bowel disease, and Eggerthella lenta, a bacteria with enzymes known to neutralize the cardiac drug digoxin. Also, species that were depleted consisted of common members of the normal gut microbiome, such as Bacteroides spp, Prevotella copri, and Alistipes shahii.6 Here are just a few examples of the plethora of findings that bring to light the association between the composition of the gut microbiome and chronic diseases.7
Of note, all of these processes also appear to be modified by the gene products and metabolites of host microbiota. For example, some clinical studies have demonstrated that supplementation with L bacillus– and Bifidobacteria-based probiotics has improved cognitive, sensory, and emotional functions in patients with Alzheimer’s disease.8 Others have used probiotics to decrease radiation- and immunotherapy-related side effects.
The Effects of Aging on Microbiota Homeostasis
Aging can influence the composition of the gut microbiome, pushing the pendulum toward a proinflammatory state and leading to gut barrier disruption and changes to the brain-gut-microbiota axis.4 It has also been postulated that specific processes during aging, such as cellular senescence, inflammation, and cancer, contribute to the overall disruption of the microbiota homeostasis. During aging, the overall microbial taxial diversity declines and may offer opportunities for newer and more dominant strains to take over the microbial environment.9 The great majority of gynecologic malignancies occur in postmenopausal women. Conceivably, a proinflammatory milieu within the aging gut, compounded by other chronic diseases such as obesity, may set the stage for and/or facilitate carcinogenesis with advancing age.
The Association Between Diet and Gut Health
Liu et al looked directly at the microbiome within the colonic mucosa and determined an association between quality of diet and the microbiome composition in human colonic mucosa.10 Rather than looking at an individual diet itself, more attention was paid to dietary patterns and their associations with colonic mucosa–associated gut microbiota. High-quality diets, rich in fruits and vegetables and low in sugar, alcohol, and solid fats, are linked to more beneficial bacteria with anti-inflammatory properties.10 Low-quality diets high in fat and sugar are associated with increases in pathogenic bacteria such as Fusobacterium, which has been shown in other studies to be linked to the development of colorectal cancer.11
The PI3K pathway indirectly links dietary intake to tumor regulation. Cells capable of evading innate and/or adaptive immune responses are selected during tumor evolution, and class I PI3K activation is necessary for efficient innate and adaptive immune system response. This allows for homeostasis and can be manipulated for antitumor activity. In fact, resident gut bacteria have been shown to activate B cells in a PI3K-dependent manner, which suppresses aggressive immune responses and maintains mucosal homeostasis.12 Furthermore, in preclinical models, a ketogenic diet was shown to decrease PI3K signaling and tumor growth.13
The PI3K/AKT/mTOR pathway has been implicated in the pathogenesis of endometrial cancer, and as such, it is being vigorously pursued as a potential therapeutic target. Endometrial cancer, in particular, is linked to numerous interconnected factors including obesity, increased inflammation, hyperinsulinemia, and changes in hormone signaling—all of which are associated with this pathway. Malignancies that use the PI3K pathway as part of its pathogenesis, such as endometrial cancer, could also serve as a rational target for microbial manipulation and immunotherapy for cancer.14
The term “estrobolome” has been defined by Plottel and Blaser as “the aggregate of enteric bacterial genes whose products are capable of metabolizing estrogens.” Intestinal microbiomes modulate the enterohepatic circulation of estrogens and their reabsorption.15 Enzymes that promote deconjugation and thus reabsorption of free estrogens may lead to an increased relative total of circulating estrogen. High levels of circulating estrogen have been linked to increased risk of hormone-driven malignancies, such as endometrial and ovarian cancers. The association between diversity of the fecal microbiome and urinary estrogen levels has been explored and indeed links the phylogenetic diversity of the gut microbiome and the ratio of metabolites to parent estrogens (E1 and E2).16 The estrobolome may prove to play a role (or roles) in estrogen receptor–associated cancers, and there are preliminary data that point to its role in breast cancer. Not yet explored is the role the estrobolome might play in modifying other hormonally responsive tumors, such as endometrial cancer and some ovarian cancers.
The Gut Microbiome and Immunotherapy for Gynecologic Cancers
The broad-reaching success of checkpoint inhibitors for varied cancer types and the concept that response to immunotherapeutics is modulated by the microbiome in our gut have sparked a great deal of interest. In one such study, Gopalakrishnan and colleagues studied microbiome samples from the oral cavity and gastrointestinal tract of patients with malignant melanoma during immune blockade treatment.17 This and subsequent studies showed that patients who responded to immunotherapy had an abundance of Clostridiales and Ruminococcaceae, the presence of anabolic pathways, and much higher infiltration by CD8-positive lymphocytes at baseline. Those without a response to immunotherapy had, in general, greater Bacteroidetes and had predominantly catabolic function.
Furthermore, Matson et al revealed eight species associated with response to immunotherapy, including Enterococcus faecium, Collinsella aerofaciens, Bifidobacterium adolescentis, Klebsiella pneumoniae, and Lactobacillus spp.18 By contrast, Ruminococcus obeum and Roseburia intestinalis were found in those who did not respond to immunotherapy.
The field of immuno-oncology has traversed to the world of gynecologic oncology, with limited but evolving current applications. Given the gut microbiome’s apparent and significant role in modulating the immune response and the body’s response to immunotherapy, ongoing studies now aim to understand the optimal bacterial milieu within the gut that best confers immuno-oncologic success. No published studies have yet evaluated the composition of the gut microbiome in those with and without a response to immunotherapy for gynecologic malignancies. However, the gut microbiome’s link to the success of radiation therapy has been explored.
Sims et al recently demonstrated that the diversity of gut microbiota may be associated with a favorable response to chemoradiation therapy in women with cervical cancer.19 Additionally, compositional variation among patients seemed to correlate with short- and long-term survival. This finding suggests that gut diversity is a significant factor for predicting overall survival in patients with cervical cancer undergoing chemoradiation therapy. Of note, the investigators analyzed immune cells from cervical tumor brush samples by flow cytometry and revealed an association between high microbial diversity, increased tumor infiltration of CD4-positive lymphocytes, and the activation of CD4 cells over the course of radiation therapy.19
Clinical Trials in Progress
At present, numerous trials are exploring how to manipulate the gut microbiota in an effort to improve clinical outcomes. Several methods are currently being investigated, including the use of prebiotics, probiotics, fecal microbiota transplantation, or capsules loaded with bacteria.
“Ongoing studies now aim to understand the optimal bacterial milieu within the gut that best confers immuno-oncologic success.”— Nathalie D. McKenzie, MD, MSPH, FACOG, DipABLM
Tweet this quote
One clinical trial (ClinicalTrials.gov identifier NCT03934827) is actively recruiting multiple solid tumors to investigate a novel biotherapeutic, MRx0518, which is composed of a proprietary strain of bacterium. Patients who have been diagnosed with one of many solid tumors and are amenable to surgical resection are eligible for this study.
Another published randomized controlled trial evaluated the use of probiotics in postsurgical colorectal cancer.20 It showed that probiotic supplementation may modify the intestinal microenvironment, resulting in a decline of proinflammatory cytokines. Whether similar interactions between immuno-oncology and the microbiome occur in gynecologic cancers has not been fully elucidated, but these studies collectively suggest that studying the interactions of oncologic therapies, diet, chronic illnesses, and malignancies merits further investigation.
Dr. McKenzie is a board-certified gynecologic oncologist at AdventHealth Cancer Institute, Orlando, Florida.
DISCLOSURE: Dr. McKenzie has served as a speaker for Clovis Oncology and Merck.
REFERENCES
1. Liu X, Zou O, Zeng B, et al: Analysis of fecal Lactobacillus community structure in patients with early rheumatoid arthritis. Curr Microbiol 67:170-176, 2013.
2. Scher JU, Sczesnak A, Longman RS, et al: Expansion of intestinal Prevotella copri correlates with enhanced susceptibility to arthritis. Elife2:e01202, 2013.
3. Brusca SB, Abramson SB, Scher JU: Microbiome and mucosal inflammation as extra-articular triggers for rheumatoid arthritis and autoimmunity. Curr Opin Rheumatol 26:101-107, 2014.
4. Kowalski K, Mulak A: Brain-gut-microbiota axis in Alzheimer’s disease. J Neurogastroenterol Motil 25:48-60, 2019.
5. Tang WWH, Kitai T, Hazen SL: Gut microbiota in cardiovascular health and disease. Circ Res 120:1183-1196, 2017.
6. Jie Z, Xia H, Zhong SL, et al: The gut microbiome in atherosclerotic cardiovascular disease. Nat Commun 8:845, 2017.
7. Dubin K, Callahan MK, Ren B, et al: Intestinal microbiome analyses identify melanoma patients at risk for checkpoint-blockade-induced colitis. Nat Commun 7:10391, 2016.
8. Mancuso C, Santangelo R: Alzheimer’s disease and gut microbiota modifications: The long way between preclinical studies and clinical evidence. Pharmacol Res 129:329-336, 2018.
9. Aleman FDD, Valenzano DR: Microbiome evolution during host aging. PLoS Pathog 15:e1007727, 2019.
10. Liu HX, Tao LL, Zhang J, et al: Difference of lower airway microbiome in bilateral protected specimen brush between lung cancer patients with unilateral lobar masses and control subjects. Int J Cancer 142:769-778, 2018.
11. Zhou Z, Chen J, Yao H, et al: Fusobacterium and colorectal cancer. Front Oncol 8:371, 2018.
12. Mishima Y, Oka A, Liu B, et al: Microbiota maintain colonic homeostasis by activating TLR2/MyD88/PI3K signaling in IL-10-producing regulatory B cells. J Clin Invest 129:3702-3716, 2019.
13. Hopkins BD, Pauli C, Du X, et al: Suppression of insulin feedback enhances the efficacy of PI3K inhibitors. Nature 560:499-503, 2018.
14. Warner AB, McQuade JL: Modifiable host factors in melanoma: Emerging evidence for obesity, diet, exercise, and the microbiome. Curr Oncol Rep 21:72, 2019.
15. Gloux K, Berteau O, El Oumami H, et al: A metagenomic β-glucuronidase uncovers a core adaptive function of the human intestinal microbiome. Proc Natl Acad Sci U S A 108(suppl 1):4539-4546, 2011.
16. Fuhrman BJ, Feigelson HS, Flores R, et al: Associations of the fecal microbiome with urinary estrogens and estrogen metabolites in postmenopausal women. J Clin Endocrinol Metab 99:4632-4640, 2014.
17. Gopalakrishnan V, Spencer CN, Nezi L, et al: Gut microbiome modulates response to anti–PD-1 immunotherapy in melanoma patients. Science 359:97-103, 2018.
18. Matson V, Fessler J, Bao R, et al: The commensal microbiome is associated with anti-PD-1 efficacy in metastatic melanoma patients. Science 359:104-108, 2018.
19. Sims TT, El Alam MB, Karpinets TV, et al: Gut microbiome diversity is an independent predictor of survival in cervical cancer patients receiving chemoradiation. medRxiv. May 22, 2020.
20. Zaharuddin L, Mokhtar NM, Muhammad Nawawi KN, et al: A randomized double-blind placebo-controlled trial of probiotics in post-surgical colorectal cancer. BMC Gastroenterol 19:131, 2019.