“They’re all charlatans,” my professor assured me when, in medical school in the mid-1970s, I expressed an interest in oncology.
The treatment of cancer with drugs, despite popular but inaccurate descriptions of its history, began in 1944 when Goodman and Gilman at Yale conducted contract research for the Department of Defense and identified the toxic effects of nitrogen mustard, an alkylating agent and active ingredient of mustard gas, on hematopoietic cells. They used the agent in patients with lymphomas and saw transient responses. Early on, progress was slow. Therapies were toxic, and little was known about supportive care. Patients were made sicker by treatment, and most of them died despite the treatment, or because of it.
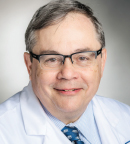
After a rocky start in the 1990s, gene therapy is becoming safer and more precisely targetable through better vectors and fail-safe genetic constructs.— Dan L. Longo, MD, MACP
Tweet this quote
Several elite chiefs of medicine (for example, from The University of Texas Southwestern, Massachusetts General Hospital, Washington University, the University of Utah, and Duke University) were actively hostile toward oncology and only reluctantly accepted oncologists into their departments. The major textbooks of internal medicine did not have an editor trained in oncology until the 1990s.
This year, 2020, will mark the 40th year in which someone other than a cancer doctor is at the helm of Dana-Farber Cancer Institute. Academic medicine had little use for oncology until cancer care became a major contributor to the financial solvency of academic medical centers.
Broadening the Palette of Targets in Oncology
The resistance to oncology as a discipline is disappearing, as treatment has become more effective, due in part to the shift from the assembly of empiric combinations of drugs to the rational design of agents aimed at specific targets in cancer cells and agents aimed to boost host defenses. New knowledge has broadened the palette of targets.
Oncology began by attacking DNA and mitotic spindles. Now we understand more about cancer cell biology, the complex interactions between cancer cells and between cancer and its host. We have recognized distinct vulnerabilities in certain cancers and have begun to exploit them. We have tools to interfere with many of the pathways activated by gain-of-function mutations in oncogenes. We have learned some of the tumor’s tricks to avoid immune detection and support itself with new vessel formation. We can overcome them. We are learning to exploit tumor defects in DNA repair and cell-cycle regulation.
Nevertheless, challenges abound. Some are based in a knowledge gap and some, in logistics.
Knowledge Gap
We do not yet have an effective tool to counter activating RAS mutations. By all estimates, an effective anti-RAS agent would be a major advancement in the treatment of many cancers. Candidates are being tested, but good news travels very fast in oncology, and we have, as yet, heard little about the clinical application of candidate RAS inhibitors.
You can’t give a person more T cells without creating room for them in the T-cell niche.— Dan L. Longo, MD, MACP
Tweet this quote
We are not yet able to replace the function of mutant tumor-suppressor genes, the big kahuna being p53. Solving that problem might well be the Rosetta stone of oncology, leading to solutions to other mysteries.
Gene Therapies and Beyond
We have seen progress in activating the immune system to recognize and kill cancer cells, both by using the passive transfer of antibodies and effector cells and by triggering intrinsic immune activation through the removal of suppressive regulation. A problem with these therapies is that, when we use them, we don’t know whether we are “turning on” a klieg light or a refrigerator light. Sometimes serious, permanent damage is done by immune activation that loses a sense of self-recognition. Much is left to learn. Only two of at least seven T-cell checkpoints have been clinically exploited to date. We are learning how to induce antigen-specific tolerance to limit toxicity and to counter an overexuberant immune response. Vaccines hold the promise of maintaining long-term antitumor immunity. The design of killer lymphocytes is being facilitated by gene therapy.
Mitochondrial energetics may be another useful target to exploit. Aerobic glycolysis seems to be the preferred pathway of energy generation by tumor cells (the Warburg effect). This pathway can be manipulated.
After a rocky start in the 1990s, gene therapy is becoming safer and more precisely targetable through better vectors and fail-safe genetic constructs. CRISPR (clustered regularly interspaced short palindromic repeats) and other gene-editing technologies may facilitate repairing specific gene mutations that influence cancer cell biology.
RNA has emerged as both a target and a tool in the fight. The role of mRNA, microRNA, and RNA splicing in cancer cell biology is shedding light on RNA as a potential anticancer target. Antisense RNA technology has already been exploited in some metabolic diseases.
Progress in treatment, as well as insights gained into resistance mechanisms, may be afforded by sequential genetic monitoring of peripheral blood circulating tumor DNA and/or cells. More insight about how tumor cells evade our therapies is essential to defining approaches to counter them.
New Ideas in Cell Biology
Additional basic science work in cell biology will also yield new ideas. Here are two examples.
First, by the time a solid tumor is clinically detectable, its growth fraction is very low (with certain exceptions like Burkitt lymphoma). Some of the cells that are not in cycle cannot re-enter it (too damaged, not enough oxygen, etc). But some of the cells are reversibly out of cycle, and, when the tumor is cytoreduced, they again become proliferative. We see this in many normal tissues. After cytotoxic chemotherapy, the marrow recovers but does not overshoot its niche. Resection of the liver leads to regeneration, with the proliferation stopping when it has reached its niche limit. You can’t give a person more T cells without creating room for them in the T-cell niche.
We are just beginning to elucidate the cell biology that controls an organ niche: the signals that make the lungs exactly the right size for the pleural cavity and the tongue the right size for the floor of the mouth. Some mechanism(s) must sense the available space and adjust to it. Evidence suggests the hippo pathway is involved, at least to some extent, in some tissues. A major issue with cancer is its disregard for its niche. It seems to mistakenly prioritize total mass over niche. When we learn how it does this, we can work to restore its responsiveness to the signals of niche limitation.
A second cellular pathway that may be exploited in cancer can be discerned by reviewing red cell maturation. As red cells mature, they gradually fill the cytoplasm with globin and the enzymes necessary to keep the cell viable for 4 months; then, the nucleus shuts down, becomes pyknotic, and is ultimately extruded to produce a mature red cell without a nucleus. How does it do this? There must be a genetic pathway that is normally “turned off,” presumably by chromatin regulation, that at just the right time becomes activated to totally shut down nuclear transcription. Wow! We could probably use that pathway, don’t you think?
I am sure there are other features of normal cell biology that are exploitable against cancer cells.
Logistics Slow Progress
As therapy becomes more effective, remissions are more common and more long-lasting. Therefore, to show that something is better, we will need more patients entered on studies and followed for longer periods; requirements that are sure to slow progress.
As more agents that have some efficacy are developed, mathematical complications arise. What combinations of agents aiming at what targets are most effective? Will combinations of three or four targeted agents be better than one or two? Is there an optimal sequence? And what if mechanistically complementary agents are made by competing companies? How can we get them to work together? And, when many agents hit the same target, how will we ever learn whether one is better than another when they are owned by different companies that may not be interested in an efficacy comparison for fear of losing the market share?
Even if we had access to the agents we want to test, putting them together must be done carefully with rigorous attention to potential synergistic toxicity as well as synergistic antitumor effects. More slowing down of the process. And, of course, the astronomical costs of new therapies are a barrier to progress.
Despite these persistent logistic and knowledge-based barriers, progress in the past 25 years has been nothing short of amazing. I, for one, have faith in my colleagues, both those with a mature understanding of where we have been and those with a vision of where we are going, to continue the rapid pace of progress that will translate the diagnosis of cancer from terror-inducing to a problem that can be overcome. ν
DISCLOSURE: Dr. Longo reported no conflicts of interest.